MicroStrategy's Significant Bitcoin Impairment Losses May Mislead: Berenberg
Turkish Crypto Exchange Thodex CEO Faruk Özer Sentenced to 11,196 Years in Prison for Collapse
DeFi and Credit Risk
Lithium-ion batteries are the world's unsung heroes. Since its commercialization in the early 1990s, its capacity to store enormous quantities of energy in a very little amount of space has revolutionized the technology sector. There would be no iPhone or Tesla, and your laptop would be more heavier and larger if lithium hadn't been invented.
When it comes to developing electric vehicles and the energy storage systems we'll need as we transition to renewables, we're running out of this valuable metal. New battery technologies are urgently sought by some of the world's leading scientists in an effort to replace lithium-ion with something cleaner, cheaper, and more abundant. Their hidden weapon might be a quantum computer.
Haber-Bosch, a century-old technology for converting nitrogen from air into ammonia-based fertilizer for crops, uses up to 5% of the world's usage of natural gas. In terms of global population support, it's critical, but inefficient when compared to nature's own ways, which can sustain around 40% of the global population. Quantum computers may be able to help.
These issues have so far been tackled with blunt instruments by researchers. The more intricate the reactions, the more difficult it is for supercomputers to manage, even if they can do more sophisticated simulations utilizing classical devices. Because of this, scientists are either able to look at a restricted number of problems, or they are compelled to compromise precision in order to meet a short-term deadline.
You can easily recreate a hydrogen atom on a laptop since it contains one positively charged proton and one electron. You can even work out its chemistry by hand. There are two protons and two electrons orbiting helium, the next step up on the periodic table, but the electrons are entangled, which means that the state of one electron is related to another, which means that all of them must be computed simultaneously.
At thulium, with 69 circling electrons, you've gone well beyond the capabilities of traditional computer systems. In order to write down every potential state of thulium every second, it would take 20 trillion years, which is more than 1,000 times the age of the universe. According to John Dowling in Schrödinger's Killer App, you'd need $600 trillion worth of Intel chips in order to mimic thulium on a classical computer if you wanted to do so.
Simply measuring the atom would be a faster alternative. Classical computers appear to decelerate exponentially while mimicking quantum systems, according to Dowling's research.
The Most Favored
on a pink backdrop, the back of a person's head wearing headphones
Listen to these 20 audiobooks right now:
By means of a WIRED EV charging lamp
If you have the financial means, bidirectional charging for electric vehicles is the way of the future.
Through the eyes of Sabrina Weiss
The Northman's Amleth, played by Alexander Skarsgrd, wears a wolf's head cap and screams.
A Cult Classic Isn't Enough for The Northman.
Will Bedingfield
Take out your wallet and take out a 50 euro note.
The Case Against Salary Adjustment Based on Geographic Location
Through the efforts of Megan Carnegie
Advertisement
That identical entangled quantum system demonstrates no exponential delay when mimicking itself. Entangled quantum systems are like computers that are tenfold more powerful than any conventional computer." Our ability to mimic chemistry hasn't been possible for more than a century because of a lack of computational capacity. The greatest strategy to dealing with complicated simulations that are intractable for classical computers is to just try a lot of different things in the actual world and derive conclusions from observation and experimentation.
At this point, "we don't know how electrons will behave," says Christopher Savoie of Zapata. "We can be more foresighted and do less actual laboratory trials if we can get to a world where we can simulate it on a computer." If you look at how Airbus is testing planes now, it looks like they're still making little plane prototypes and tossing them into the air. As Google's Sergio Boixo explains, "You cannot imitate molecular processes that you are interested in." As far as low-level materials science and engineering, you're essentially blind. "
When it comes to solving a wide range of complex scientific issues like these, scientists need computers with quantum components, just like Richard Feynman predicted back in the 1980s. According to Boixo's Google colleague Marissa Giustina, you may conceive of a quantum computer as a programmable molecule. "Like a molecule, it's a complex system behaving according to the laws of quantum physics. As a result, you may envision a way to get from there to programming chemistry."
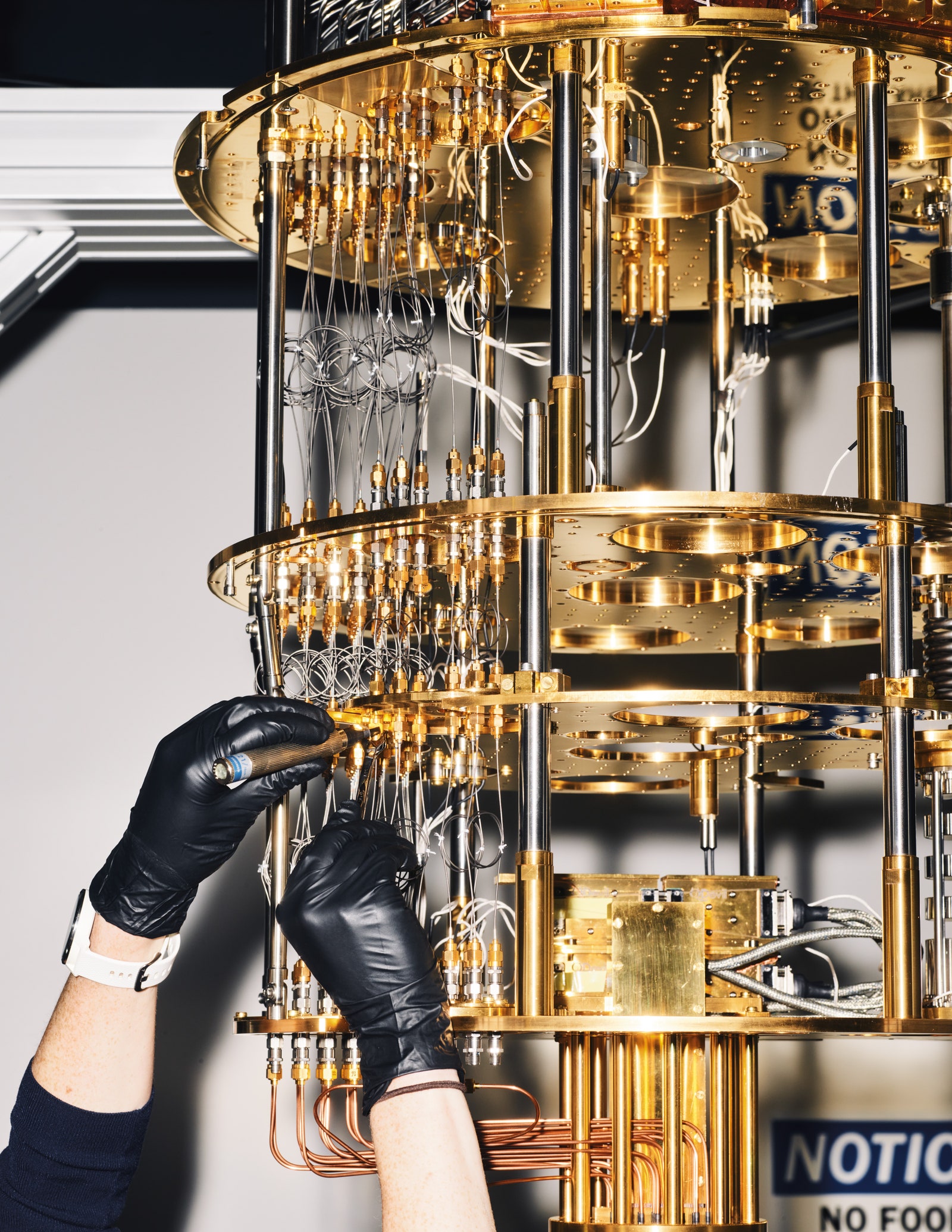
ZAPATA co-founder and chemistry professor ALN ASPURU-GUZIK worked with University of Melbourne quantum physicist Andrew White and colleagues in 2010 to undertake one of the first quantum chemical simulations. They chose dihydrogen, which is a simple molecule that would not present any difficulties to a classical computer or even to a scientist using a pen and paper. They chose dihydrogen.
In 1927, the then-new science of quantum mechanics was used to analyze dihydrogen, which is merely two hydrogen atoms bonded together. As a proof-of-concept, it was sufficient to demonstrate that quantum computers could do this type of calculation. As precise as six parts per million, their quantum simulation, which used a photon-based quantum gadget, could determine the strength of the hydrogen-atom link.
It is possible to use quantum computers to increase our knowledge of chemical processes in three ways: It is possible to develop a computer model of the problem you're attempting to solve by physically reproducing the exact structure of the molecule with the correct amount of qubits. Even if you were to develop a rudimentary machine that could be reprogrammed to solve different tasks, it would not be a computer in the classic sense.
Algorithms that explain how a system evolves over time are used in the second method. Watch the system's wave function and Hamiltonian evolve over time as you enter these variables. There are a wide range of applications for these 'Hamiltonian simulations,' as they're commonly referred to, and they might be particularly valuable when it comes to complicated processes in which the electrons are coupled.
Quantum computers offer an exponential speed-up in a number of active challenges, such as this one. For example, catalytic metal extraction and carbon dioxide fixation are only two chemistry problems that could be solved by a quantum computer powerful enough and trustworthy enough. It's possible that fertilizer production might have the greatest influence, though. Nitrogen is essential to the growth of plants. Because plants are unable to draw nitrogen from the sky, farmers must supplement their crops with nitrogen-rich fertiliser created using the energy-intensive Haber–Bosch process. A loaf of bread's carbon footprint is 40% due to the nitrogen needed to produce the fertilizer used to grow wheat.
Nature, on the other hand, has its own way of doing things. A bacterial enzyme known as nitrogenase is used by some plants to 'fix' nitrogen from the air and turn it into ammonia. The Haber-Bosch process and the development of less energy-intensive synthetic fertilizers might both benefit from a better understanding of how this enzyme functions.
FeMoco, a molecule at the center of the enzyme that is too complicated for traditional computers to represent, is the key to fixing that challenge. An ETH Zurich-Microsoft team showed in 2017 that a quantum computer with one million physical qubits may solve this challenge, although the researchers also admitted that it would require millions of logical ones as well.
Furthermore, it is possible that Hamiltonian simulations might be used to better understand how plants utilize the sun's energy to grow. Photosystem II is a massive, multi-enzyme system found in plants that is responsible for many of the initial processes in photosynthesis. An artificial photosynthesis technology based on quantum computers might let chemists harness the sun's power to produce fuel.
By speeding up the hunt for novel materials for solar panels, quantum computers might be of use. New materials for batteries and superconductors that function at normal temperature might also be discovered using this method, which could lead to new developments in the motors, magnets and quantum computers themselves.
New materials can be discovered by using generative modeling in the same way that data for machine learning can be generated from a limited amount of real-world data. In order to build comparable items, "we may utilize generative modeling if we have a hundred samples," Savoie explains. In order to identify novel compounds, we can conduct screening of chemical libraries, or we can develop virtual chemical libraries.
Because quantum computers may be able to discover novel substances, the medical industry is enthusiastic about the technology. MRI scan data can be processed more efficiently and precisely by quantum computers, but they might also save billions of dollars in medication discovery by allowing corporations to swiftly find novel chemicals and then mimic their effects without having to synthesize them. Scientists might use quantum computing to develop novel therapies for diseases like Alzheimer's or to better understand diseases like Covid-19, which are still in their infancy. Quantum computers will speed up the process of discovering how proteins fold, a fundamental component of growth and illness.
A quantum computer with hundreds or millions of qubits may be required for most of these applications, but some natural problems that were previously inaccessible might be solved within the next decade, according to several experts in the area. As a matter of fact, it may be advantageous for quantum computers to be noisy and error-prone in their early stages of development since the real world is a loud place, too.
"For many applications of quantum devices, such as encryption, this noise can be a significant constraint and lead to intolerable levels of mistake," write Anton Toutov and Prineha Narang in an essay for WIRED. This is not the case while simulating chemistry because the noise in such simulations would be indicative of a molecule's (or other chemical system's) actual environment. Although NISQ simulations of molecules will be noisy, this noise actually offers you significant information about the molecule's natural surroundings."
Rather than a flaw, noise and flaws in nature simulations might be considered an asset. Chemistry and materials research are already benefiting from the usage of miniature quantum computers equipped with resource-saving algorithms that are already being developed.
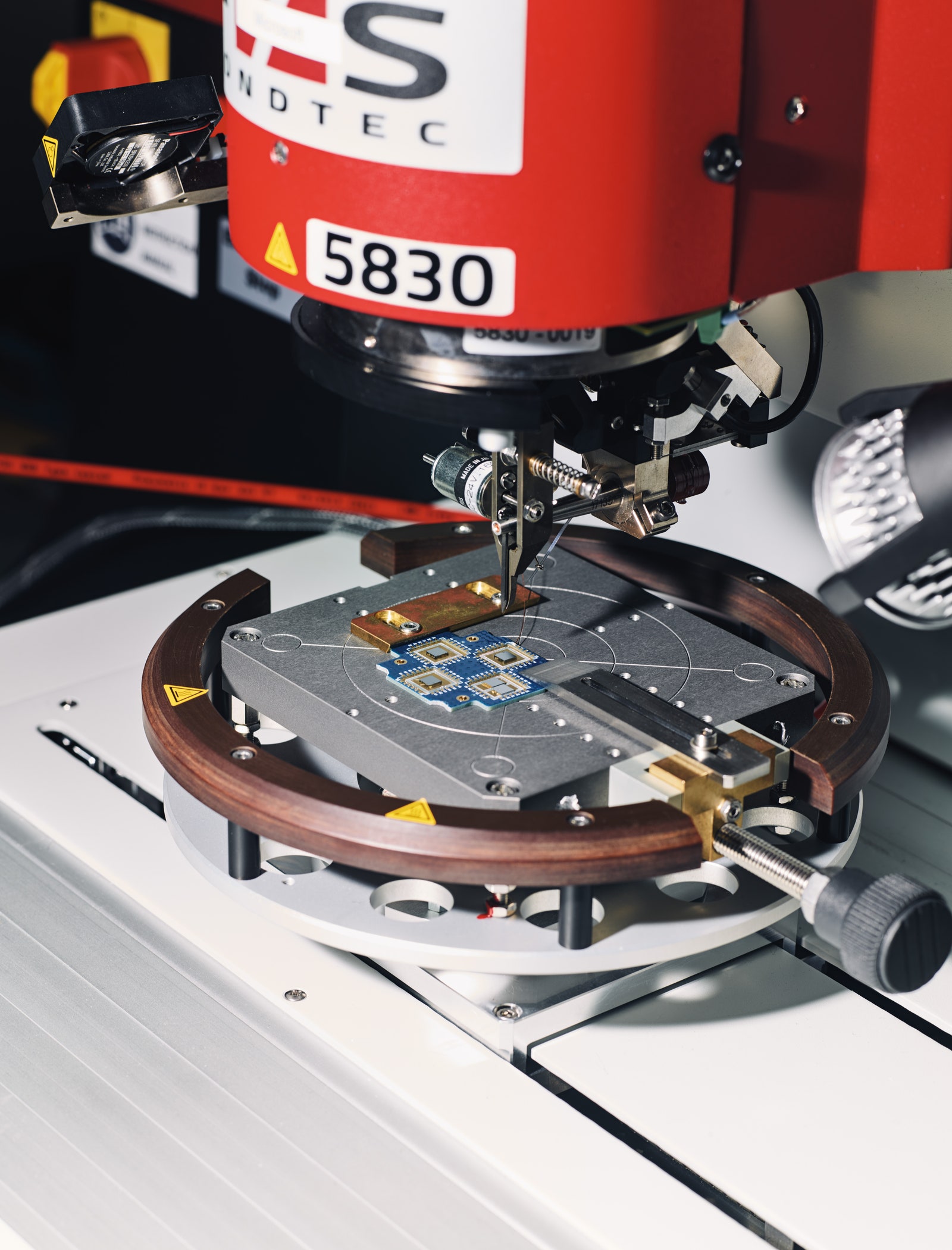
An early look of how quantum computers may be beneficial in the NISQ era was published by IBM researchers IN JANUARURY 2020. A small-scale quantum computer was used to model the behavior of three molecules containing lithium, which might be employed in the next generation of lithium-sulphur batteries that promise to be more powerful and less expensive than today's power cells. Because they didn't have enough qubits to execute a Hamiltonian simulation, they instead employed variational quantum algorithms, which is the third way quantum computers may replicate nature and is expected to be the most effective for short and medium term applications.
Variational quantum algorithms employ a combination of quantum and conventional computers in order to speed up computations. ' Google Maps' optimal route home in a reasonable period of time is likened by Zapata's principal research scientist and founder Peter Johnson in a blog post. As he points out, the program does not look for all viable routes. This instead results in a well-intentioned subset and partial route search." Google's mapping system employs shortcuts and rules of thumb to reduce the amount of the database it searches through, according to Johnson.
On an unknown street where the odd and even numbers are separated by a few feet, you may utilize this strategy to find the home number you're searching for. You can cut your search time in half by only looking on one side of the road, with negligible effect on the end outcome.
To avoid wasting time and resources on a whole computation, variational quantum algorithms can instead employ a small number of qubits to make a best guess at the answer and then give the result over to a conventional computer, which then determines whether to try again. Using fewer, noisier qubits is possible because the quantum computation is divided into smaller, independent processes.
A 'variational quantum eigensolver' technique and Google's superconducting qubits were used by Zapata's Alán Aspuru-Guzik and the Santa Barbara team at Google in 2016 to simulate dihydrogen once more.
It was once again possible for a quantum computer to anticipate the molecule's energy states and bond lengths. As the complexity of systems grows, the technique's potential of simpler scaling up to more complex systems grows as well.
One of the things you can do with the variational quantum eigensolver is discover the minimal energy of your issue," explains IBM's Heike Riel. It's typical to have an equation that characterizes your physical system, and one difficulty to address is determining the lowest energy of this equation. These qubits can be used in a wide range of applications from optimization problems like the traveling salesman, to chemical reactions where you need to find the ground state (the lowest possible energy level of a system), and ones where an excited state (any other energy level) is of interest, as is the case with photosynthesis and solar energy.
It's becoming easier for the public to have access to early quantum computers' massive amounts of qubits. There is IBM's Q network and there is Microsoft's Azure cloud computing platform that includes quantum devices. In the next few years, researchers in the domains of chemistry and biology might witness some early benefits of quantum computing by combining these platforms with quantum-inspired optimisation algorithms and variable quantum algorithms. Some of the most pressing challenges confronting our world may one day be solved by quantum computers, according to Google's Sergio Boixo. It's "an energy problem," he explains, because "energy is a physical, chemical process."
"Perhaps we can create a new industrial revolution that uses energy more efficiently if we provide the tools that allow simulations to be done." But in the end, quantum computers may have the greatest influence on quantum physics.
While smashing protons together in an attempt to discover the basic secrets of the universe, the Large Hadron Collider, the world's biggest particle accelerator, captures 300 gigabytes of data per second. There are already 170 data centers spread across 42 countries that are being used to analyze it. They anticipate that quantum computers might help speed up data processing by allowing researchers to perform more precise simulations before conducting real-world testing at CERN (the European Organization for Nuclear Research). When quantum computers are smart enough to help, they're starting to design algorithms and models to help them harness their potential.
CERN scientist Federico Carminati told WIRED in 2019: "Even though we are late to the game, we are offering unique knowledge in several disciplines." "These are our first efforts in quantum computing." "Quantum computing is based on our expertise in quantum physics." The 2012 discovery of the Higgs boson, an elementary particle whose existence confirmed several long-held but evidence-light ideas of quantum physics, is the Large Hadron Collider's most significant achievement to date.
Scientists from Caltech and the University of Southern California used quantum computers in 2018 to reproduce the results of their previous analysis of the data that led to that discovery. Despite the fact that it wasn't faster than a conventional machine, it indicated that a quantum machine might be employed for that sort of issue. For example, Carminati noted that "a quantum computer – itself a quantum system" may be used to produce extremely precise simulations of quantum systems. Big data analysis will be made possible by combining quantum computing with artificial intelligence, which is an ambitious yet necessary project right now.
If not the most significant, then certainly the most profound use of quantum computers, John Gribbin argues in Computing with Quantum Cats. In order to come up with a "theory of everything" that incorporates quantum theory and gravity, "it is very probable that it will only be found with the assistance of quantum computers to model the behavior of the universe," he adds. "
=====
Related Video: